Alright, you’re about to take a wild dive into the future of tech. No boring intros, no fluff—just straight-up, what the heck is quantum computing and why should you care? You might think computers already do everything we need, right? Well, think again. Quantum computing isn’t just faster; it’s an entirely different beast. We’re talking about computers that can make your souped-up gaming rig look like an abacus. Intrigued? Good, let’s jump in.
How Quantum Computing Is Different from Classical Computing
So, you know your regular computers—laptops, desktops, that pocket-sized marvel called your smartphone—all work with bits, right? Bits are either a 0 or a 1. They’re binary, simple, clean, and predictable. However, quantum computing takes this simplicity and turns it upside down. In quantum computing, bits turn into quantum bits, or qubits. Unlike classical bits, qubits can be a 0, a 1, or even, both at the same time. This phenomenon is known as superposition.
Superposition allows qubits to exist in multiple states simultaneously. This is a game-changer because it enables quantum computers to process a vast number of possibilities all at once. Imagine your laptop trying to solve a complex puzzle; it does it step by step. A quantum computer, on the other hand, uses superposition to explore multiple solutions at the same time, making it exponentially faster.
Moreover, quantum computers also use another mind-boggling feature called entanglement. When qubits become entangled, the state of one qubit is directly related to the state of another, no matter how far apart they are. This interconnectedness, combined with superposition, is what gives quantum computers their remarkable power. Theoretically, they can solve problems that would take classical computers millions of years, all before you finish your breakfast.
The Potential Impact of Quantum Technologies on Industry
Quantum computing is poised to hit industry like a thunderbolt—from cryptography to complex problem-solving, we’re talking revolution, not evolution. Imagine trying to crack an encryption code that, today, would take thousands of years. With quantum computing, this could be done in mere minutes. The implications are staggering, especially for industries that rely on secure transactions and data handling.
Banking, for example, could see a complete overhaul of encryption and security protocols, making transactions safer and faster. Logistics companies might optimize their routes in ways that classical computing simply can’t match, leading to cost reductions and improved efficiency. Healthcare could also benefit immensely, from simulating complex biological systems to discovering new drugs more rapidly.
If you thought the internet was transformative, just wait. Quantum technologies are set to redefine what is possible, opening doors to advancements that were previously only theoretical.
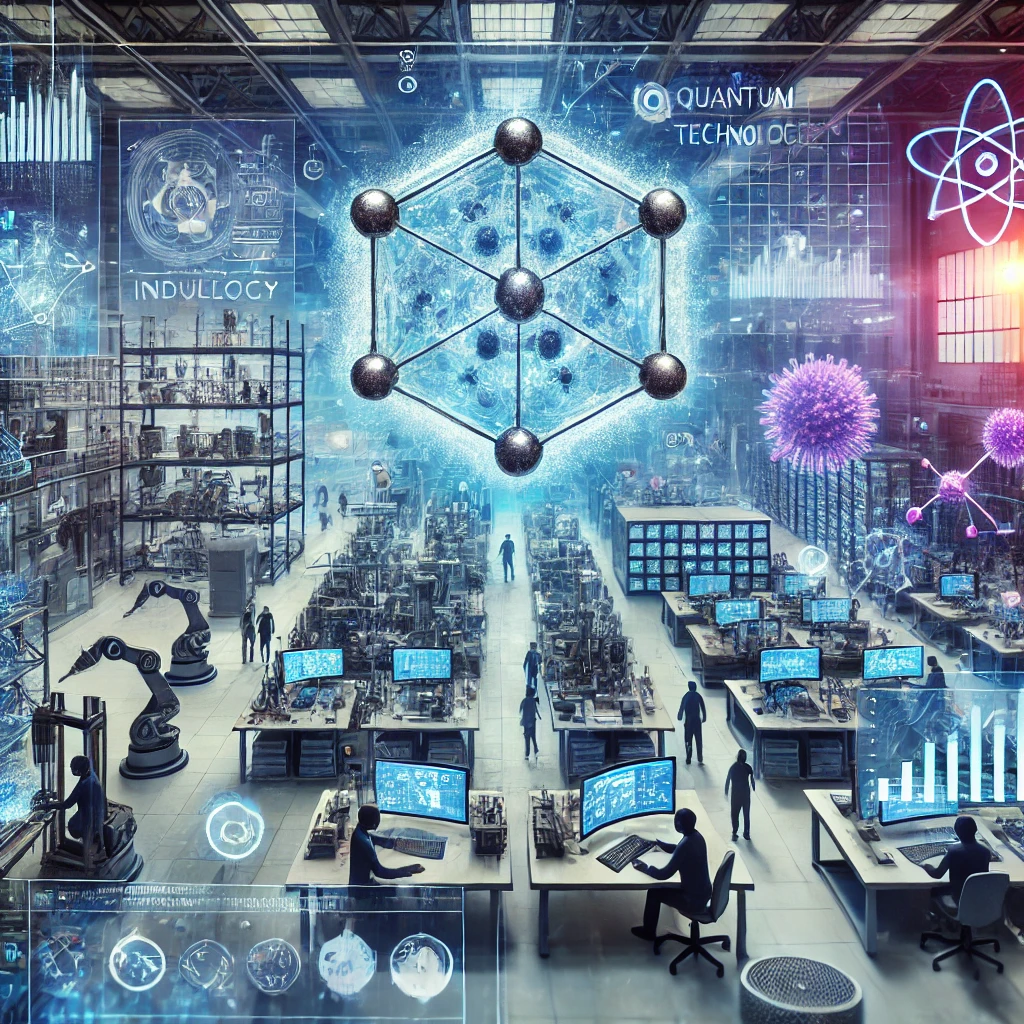
Quantum Mechanics: The Foundation of Quantum Computing
To understand quantum computing, you need to grasp a bit about quantum mechanics. However, trust me, this isn’t like your high school physics class where everything followed predictable rules. Quantum mechanics is full of surprises and unpredictability.
Consider Schrödinger’s cat—a famous thought experiment where a cat is both dead and alive until observed. This idea captures the strange, dual nature of quantum particles. Then there’s entanglement, where particles influence each other instantaneously, even if they’re separated by light-years. It’s mind-boggling, yet this interconnectedness forms a core part of quantum computing.
Moreover, quantum mechanics tells us that just observing a particle can change its behavior. This phenomenon, known as the observer effect, underpins much of what makes quantum computing possible. It may sound bizarre, but it provides the most accurate framework we have for explaining the universe at its tiniest scales.
What Is Quantum Mechanics?
Quantum mechanics deals with particles that are much smaller than atoms. These particles behave in ways that defy our everyday experiences. For instance, a particle can exist in multiple places at once until it is observed. This phenomenon is known as superposition, and it’s as strange as it sounds.
Moreover, quantum particles also exhibit a behavior called entanglement. When particles are entangled, their states become linked, meaning that a change in one instantly affects the other, regardless of distance. This kind of interconnectedness is fundamental to the power of quantum computing.
Additionally, quantum mechanics introduces us to the concept of wave-particle duality. Essentially, particles can behave both like waves and like solid particles, depending on how we observe them. These quirky behaviors form the basis for the incredible capabilities of quantum computers, allowing them to solve complex problems much faster than classical computers.
Key Principles: Superposition, Entanglement, and Uncertainty
Superposition, entanglement, and uncertainty—these are the cornerstones of quantum mechanics. Superposition allows qubits to exist in multiple states simultaneously. This means a qubit can be both 0 and 1 at the same time, which gives quantum computers their incredible parallel processing power.
Entanglement, on the other hand, is a phenomenon where two qubits become so interconnected that the state of one directly affects the other, regardless of the distance between them. This unique feature enables quantum computers to solve complex problems by working with entangled qubits that communicate instantaneously, which is impossible in classical computing.
Finally, uncertainty, often represented by Heisenberg’s uncertainty principle, suggests that we cannot precisely know all properties of a particle simultaneously. This unpredictability is not just weird—it’s fundamental to the quantum world. Together, these principles give quantum computing its mysterious yet powerful edge over classical systems.
How Quantum Processors Differ from Classical Processors
Classical processors are like the tortoises of computation—solid, dependable, but a bit slow for today’s mega-complex challenges. They are reliable but can’t handle massive computations in a short time. Quantum processors, on the other hand, are like hares on a Red Bull-fueled rampage, moving at astonishing speeds.
Quantum processors use qubits instead of traditional bits. Qubits can represent both 0 and 1 at the same time, thanks to a phenomenon called superposition. This feature allows quantum processors to evaluate many possibilities simultaneously, significantly boosting processing power.
Moreover, quantum processors harness the concept of entanglement, where the state of one qubit directly influences another, regardless of distance. This entangled state enables quantum processors to perform complex calculations far more efficiently than classical ones, making them appear almost ancient in comparison.
Qubits vs. Classical Bits: A Comparison
Classical bits are like dependable friends who always follow the rules—either they are on (1) or off (0). They are straightforward and predictable, making them easy to understand and control. However, qubits are more like that unpredictable buddy who could be anywhere between ‘on’ and ‘off.’ In fact, they can even be both at the same time, thanks to a phenomenon called superposition.
This ability to exist in multiple states simultaneously gives qubits incredible power. They are not restricted by binary choices, which allows them to explore many solutions at once. Unlike classical bits, qubits leverage quantum properties, such as superposition and entanglement, to solve problems that are impossible for classical bits.
Moreover, qubits can become entangled, meaning their states are interdependent, even over great distances. This property allows quantum computers to perform complex computations far more efficiently than any classical system could ever hope to achieve.
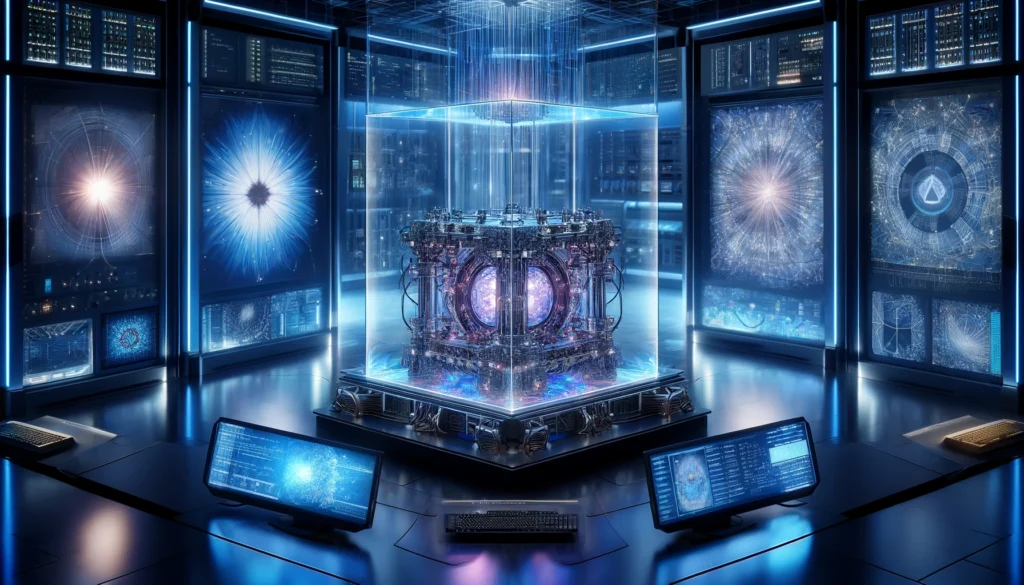
The Concept of Quantum Speedup and Quantum Parallelism
Quantum speedup isn’t just about doing the same thing faster—it’s about doing things differently. Unlike classical computers, which process tasks sequentially, quantum computers can tackle multiple possibilities simultaneously. This is what makes quantum computing so transformative.
Quantum parallelism means a quantum computer can evaluate numerous possibilities at once. This ability is incredibly powerful, especially for problems involving significant guesswork or numerous combinations. For instance, imagine trying to break encryption codes or solve complex optimization puzzles. Quantum computers can evaluate all potential solutions simultaneously, reducing processing times from years to minutes.
Moreover, quantum speedup doesn’t just offer incremental improvement; it offers exponential advances. Problems that would take classical computers decades to solve can now be handled swiftly. As a result, industries such as cryptography, logistics, and pharmaceuticals are on the brink of significant breakthroughs, all thanks to the unique power of quantum parallelism.
What Are Quantum Algorithms and How Do They Work?
You’re probably thinking, “Great, so quantum computers are different, but how do they actually do anything?” That’s where quantum algorithms come in. Traditional algorithms are step-by-step recipes to solve problems. Quantum algorithms, though, leverage all that funky quantum behavior—superposition, entanglement, and more—to sift through data and find solutions faster than classical algorithms ever could.
Quantum Algorithms: Leveraging Quantum Properties
They use principles like superposition and entanglement to solve problems efficiently. Take Grover’s algorithm, for instance—it’s all about using quantum states to sift through unsorted data.
Imagine trying to find a needle in a haystack. Grover’s algorithm does this without looking at every single straw, drastically reducing search times. Classical algorithms would require a linear search, but Grover’s approach uses quantum superposition to evaluate multiple possibilities simultaneously.
Moreover, Grover’s algorithm shows the true potential of quantum speedup. It can search through a list of N items in roughly the square root of N steps. This level of efficiency is game-changing for tasks like database searches and optimization problems. By leveraging quantum properties, Grover’s algorithm provides a significant speed advantage over classical approaches, making it a standout in the quantum world.
The Importance of Quantum Algorithms for Complex Problem-Solving
Ever tried factoring a huge number? Sure, you could do it with a classical computer, but it would take an extremely long time. Quantum algorithms, such as Shor’s algorithm, are specifically designed for these challenging tasks. Shor’s algorithm leverages quantum properties like superposition and entanglement to find factors efficiently, which classical computers struggle to achieve.
For instance, factoring large numbers is a fundamental issue in cryptography. Classical computers would need years to solve it, but quantum algorithms can do it in minutes. This ability to tackle such complex mathematical problems makes quantum computing crucial for the future of data security.
Moreover, quantum algorithms don’t just speed up existing processes—they transform them. They break down tasks into manageable units, which classical computers find impractical. This capability is why fields like cryptography, simulations, and optimization have so much to gain from quantum advances.
Key Quantum Algorithms and Their Significance
There are some heavy hitters in the quantum algorithm world that deserve a mention. Shor’s algorithm, for example, can factor large numbers super quickly—a potential nightmare for current encryption methods. Then there’s Grover’s algorithm, which drastically reduces the time needed to search through huge datasets.
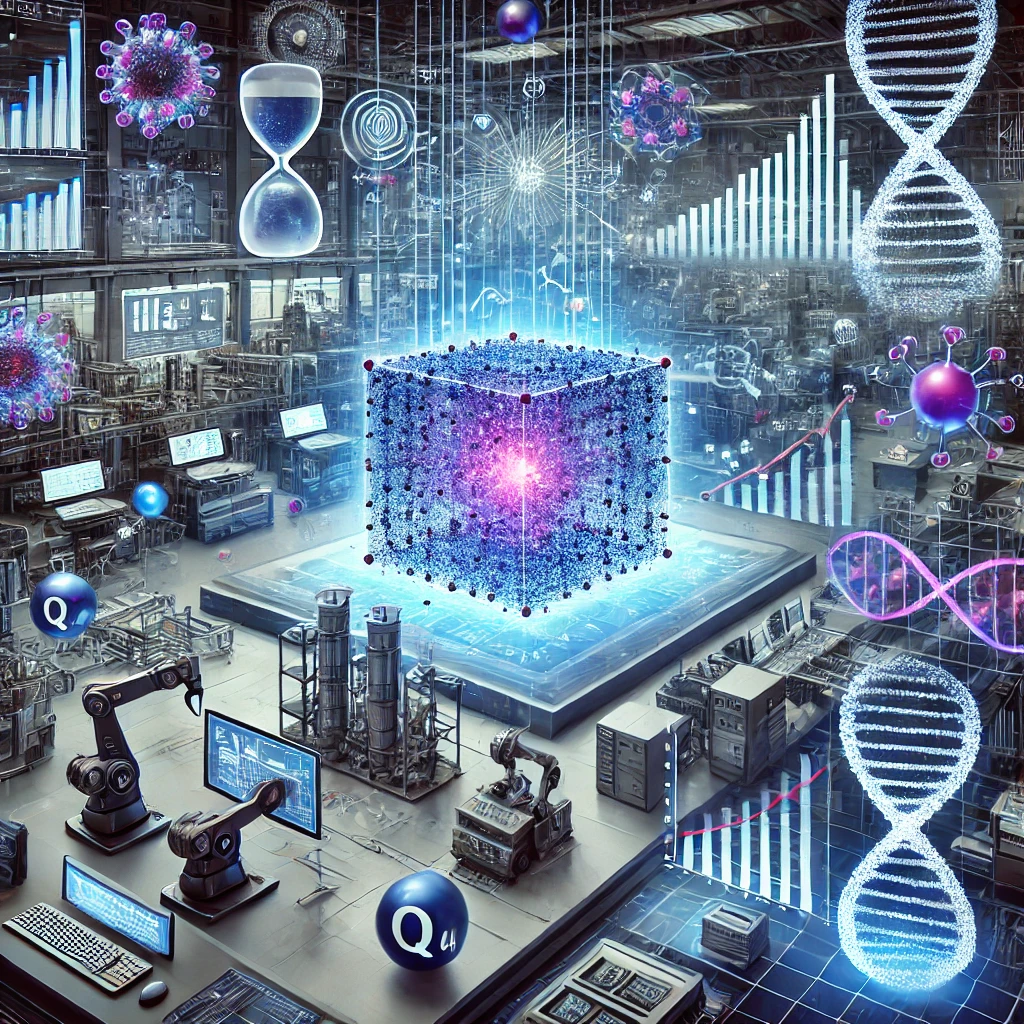
Shor’s Algorithm for Factoring Large Numbers
This one’s famous because it makes current encryption methods like RSA look like child’s play. Shor’s algorithm takes advantage of quantum computing’s ability to test multiple possibilities simultaneously, rather than one at a time. This parallelism allows it to efficiently find the factors of large numbers, which classical computers struggle with.
In traditional computing, factoring large numbers is a time-consuming and resource-intensive task, often taking years. However, Shor’s algorithm dramatically shortens this timeframe, reducing it to mere minutes. This capability poses a significant threat to encryption methods like RSA, which rely on the difficulty of factoring large numbers for security.
Moreover, Shor’s algorithm exemplifies the disruptive potential of quantum computing. It highlights why quantum supremacy, the point where quantum computers outperform classical ones, is such a game-changing concept. With Shor’s algorithm, quantum computing demonstrates its ability to solve real-world problems that were once thought intractable.
Grover’s Algorithm for Database Search
Instead of searching through a database item by item, Grover’s algorithm can find what you need in roughly the square root of N time. This speed is far greater than any classical method currently available, and it significantly improves search efficiency.
Think of it this way: classical methods are like searching for a needle in a haystack by going through each piece of hay one by one. In contrast, Grover’s algorithm is like using a giant searchlight that instantly illuminates the needle, making it immediately visible.
Moreover, Grover’s algorithm exemplifies the unique power of quantum computing. By using superposition, it evaluates multiple possibilities simultaneously, cutting down on search times dramatically. This advantage makes Grover’s algorithm particularly useful for large databases and complex problems, offering a clear showcase of how quantum computing surpasses classical limitations.
Quantum Entanglement and Superposition: The Magic Behind Qubits
Entanglement and superposition aren’t just buzzwords—they’re the secret sauce of quantum computing. Without them, qubits would just be glorified classical bits. These properties are what give quantum computing its unique power, allowing for a level of connectivity and parallel processing that’s otherwise impossible.
How Entanglement Allows Qubits to Be Interconnected
Imagine two qubits, one on Earth and one on Mars. If they’re entangled, changing the state of one instantly changes the state of the other, no matter the distance between them. This phenomenon is called quantum entanglement and forms the backbone of quantum communication.
Einstein famously called it “spooky action at a distance” because it defies classical physics and common sense. It is indeed strange to think that two particles could communicate instantaneously, even across vast distances. Yet, entanglement is real and has been experimentally verified countless times.
Furthermore, this property of qubits is what makes quantum computing so powerful. By entangling multiple qubits, quantum computers can solve complex problems much faster than classical computers. It allows for the transmission of information in a way that classical computers simply cannot replicate, offering the potential for ultra-secure communication channels and parallel processing capabilities.
The Role of Superposition in Enabling Parallel Computations
Superposition is why quantum computers can try multiple pathways simultaneously, rather than one after another. This phenomenon allows qubits to exist in multiple states at once, exploring various outcomes concurrently. It’s like having multiple brains, each tackling different parts of a problem, all in the same moment.
Imagine trying to solve a jigsaw puzzle. A classical computer tries each piece, one at a time, until it fits. A quantum computer, leveraging superposition, tries multiple pieces simultaneously, significantly speeding up the entire process. This parallel processing power is what gives quantum computing its edge over classical systems.
Moreover, superposition is a fundamental reason why quantum computers have enormous potential speedup. It lets them tackle complex challenges, like factoring large numbers or optimizing logistical routes, in ways classical computers simply cannot match. The result is a dramatic reduction in processing time for specific, complex tasks.
Quantum Gates and Quantum Circuits: Building Blocks of Quantum Computing
Just as classical computers use logic gates to perform operations, quantum computers use quantum gates. These gates manipulate qubits, bringing about the kind of computations we need for practical uses.
Understanding Quantum Gates Like Hadamard, Pauli-X, and CNOT
The Hadamard gate takes a qubit and puts it into superposition, creating an equal mix of 0 and 1. This superposition is fundamental for quantum parallelism, allowing quantum computers to evaluate multiple possibilities simultaneously. It essentially gives the qubit a 50-50 chance of being 0 or 1, creating a state that is a combination of both.
The Pauli-X gate, on the other hand, acts like a quantum NOT operation. It flips the value of a qubit from 0 to 1 or from 1 to 0. This gate is crucial for changing the state of a qubit during computation, enabling dynamic manipulation of quantum data.
The CNOT gate, or controlled-NOT gate, is where things get particularly interesting. It can flip one qubit’s value based on the state of another, which is key to creating quantum entanglement. Entanglement is vital for quantum algorithms that require qubits to work together in a coordinated way.
How Quantum Circuits Are Created and Used to Implement Algorithms
Quantum circuits are collections of quantum gates, designed to achieve a particular task. You line up the qubits, apply a sequence of gates, and—voilà—you’ve got quantum magic happening, solving problems far faster than your average computer.
Applications of Quantum Computing: Solving Complex Problems
Now, you might be wondering—great, but what can quantum computers actually do? The answer is: a lot. From cryptography and drug discovery to solving optimization problems that would take classical computers decades, quantum computing is like unlocking cheat codes for reality.
Quantum Computing in Cryptography and Security
Modern cryptography relies on the fact that classical computers are just too slow to crack codes. Encryption methods like RSA work because classical computers would take years to factor large numbers. However, quantum computers, especially with Shor’s algorithm, change the game entirely. Shor’s algorithm can efficiently factor these large numbers, making current encryption vulnerable.
This is why the development of post-quantum cryptography is such a hot topic right now. Experts are racing to create encryption methods that are resistant to quantum attacks. The urgency is clear: quantum computers threaten the security infrastructure that underpins our digital world.
Moreover, industries like banking and government are particularly concerned. They rely heavily on secure data transmission, and quantum computing could undermine this. Therefore, research into quantum-resistant cryptography is not just important, it is critical to safeguarding future digital security.
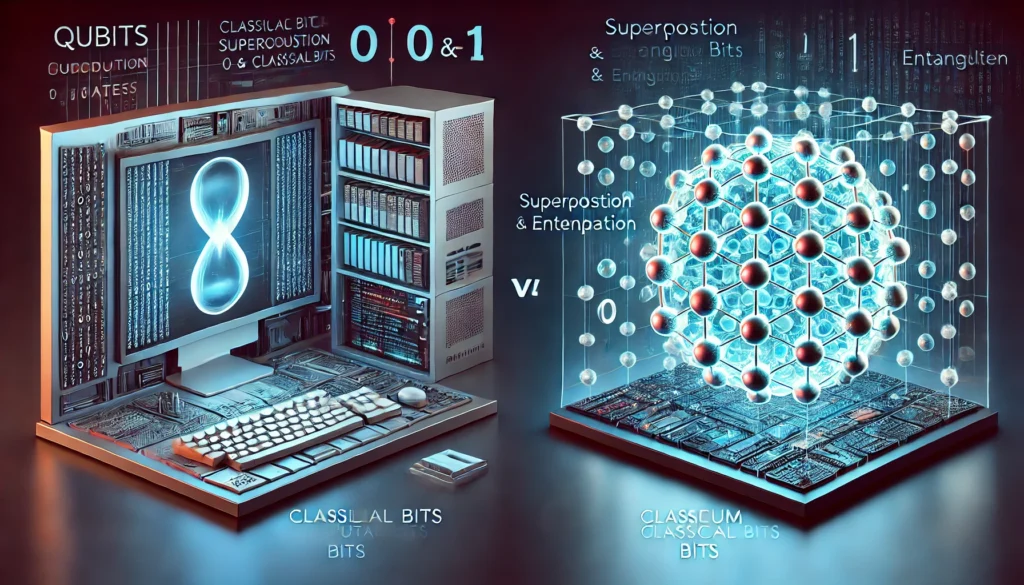
Applications in Optimization, Material Science, and Drug Discovery
Whether it’s optimizing delivery routes, simulating new materials, or searching for the next wonder drug, quantum computers can handle these challenges with incredible efficiency. Unlike classical systems, which process tasks sequentially, quantum computers operate using quantum properties like superposition and entanglement. These properties enable them to explore numerous solutions simultaneously.
For example, logistics companies can use quantum computing to optimize delivery routes in real-time. This results in cost reductions and significant improvements in efficiency. Similarly, pharmaceutical researchers can accelerate drug discovery by simulating molecular interactions more accurately than classical computers can.
Furthermore, quantum computing’s ability to simulate new materials helps scientists develop advanced materials for industries like aerospace and energy. These applications showcase how quantum computing can revolutionize fields that rely on complex problem-solving. It’s not just about speed—it’s about solving problems that classical computers could never realistically tackle.
Challenges and Limitations of Current Processors for Quantum Computing
It’s not all rainbows and superposition, though. Current quantum processors have some serious limitations—mainly around error correction and scalability.
Quantum Error Correction and Decoherence
Quantum bits are notoriously unstable and sensitive to their environment. Even a tiny disturbance can cause a qubit to decohere, which means it loses its delicate quantum state. This loss, called decoherence, makes quantum computation extremely challenging. Maintaining the integrity of qubits requires isolating them from noise and external influences, which is not easy.
Quantum error correction is the specialized field dedicated to preventing and fixing these errors. Unlike classical bits, which are either 0 or 1, qubits can exist in superpositions, making error correction far more complex. The process involves encoding information across multiple qubits to detect and correct errors without collapsing their quantum states.
However, quantum error correction remains a significant technical hurdle. The need for numerous physical qubits to create a single logical, error-free qubit makes scaling quantum computers challenging. Yet, advances in this area are crucial for the future of reliable quantum computing.
Technical Challenges in Scaling Processors for Quantum Computing
Scaling quantum processors is like trying to build a card tower on a moving table—really tricky. Quantum systems are highly sensitive to environmental factors, and even the slightest disturbance can disrupt coherence. Maintaining coherence across a large number of qubits is therefore a major challenge.
Moreover, minimizing noise is equally important but incredibly difficult. Quantum noise can lead to errors in computations, which makes scaling up quantum technology an intricate problem. Effective error correction methods are essential to keep the qubits stable, yet developing such systems is far from simple.
These challenges are compounded by the need for extremely low temperatures and specialized equipment. Quantum processors require supercooled environments to reduce thermal noise. As a result, building a large-scale quantum computer involves overcoming technical hurdles in hardware, software, and quantum error correction—all while keeping the qubits stable enough to perform meaningful calculations.
FAQs about Quantum Computing
What is quantum computing and how does it work?
Quantum computing is a type of computation that takes advantage of quantum mechanics principles like superposition and entanglement to perform calculations at speeds.
How do quantum processors differ from traditional processors?
Quantum processors use qubits that can exist in multiple states at once, while classical processors use bits that are either 0 or 1.
What are qubits and how are they used in quantum computing?
Qubits are the fundamental units of information in quantum computing.
What is quantum entanglement and why is it important?
Quantum entanglement is a phenomenon where two or more qubits become interconnected in such a way that the state of one instantly affects the state of the other, regardless of the distance between them.
How do quantum algorithms differ from classical algorithms?
Quantum algorithms leverage quantum properties like superposition and entanglement to solve problems more efficiently than classical algorithms.
What are some examples of quantum algorithms?
Some key quantum algorithms include Shor’s algorithm, which can factor large numbers efficiently (posing a threat to many encryption schemes).
What are the main challenges in building quantum computers?
One of the biggest challenges in building quantum computers is maintaining the stability of qubits.
How do quantum gates work in a quantum circuit?
Quantum gates are the building blocks of quantum circuits.
What are the real-world applications of quantum computing?
Quantum computing has a wide range of potential applications, including cryptography, optimization, material science, drug discovery, and solving complex logistical problems.
What is the future potential of quantum algorithms?
The future of quantum algorithms is bright, with ongoing advancements that promise to revolutionize data analysis, secure communications, and complex simulations.
The Future of Quantum Algorithms and Quantum Computing
We’re at the beginning of what’s sure to be a wild ride. As quantum algorithms improve, and as we figure out how to stabilize qubits and scale processors, the applications will explode. Imagine near-instantaneous data analysis, perfectly secure communications, and new technologies we haven’t even dreamed of yet.
Quantum computing may still be in its infancy, but its potential—oh man, it’s massive. The foundation we’re laying now could change the world in ways we can’t yet predict. Stay tuned, because quantum computing is only just getting started, and you wouldn’t want to blink and miss it.