Alright, let’s dive into the latest and greatest from the world of quantum physics—because, honestly, who doesn’t want to understand how the building blocks of reality might be reshaping? This isn’t just about complex equations; it’s about grasping the very essence of the universe. Recent breakthroughs in quantum physics are giving us a peek into the very fabric of existence. Buckle up, because this is going to be a ride through quantum particles, entanglement, and the fundamental principles of reality.
The Latest Experiments and Discoveries in Quantum Physics
Recent breakthroughs in quantum physics have been nothing short of transformative. Experiments with quantum entanglement and superposition are pushing our understanding of reality to new limits. Quantum mechanics, particularly through developments in quantum computing and quantum field theory, has allowed us to verify phenomena that were once purely theoretical.
For example, experiments with the Large Hadron Collider (LHC) have shed new light on quantum field interactions, expanding our understanding of the fundamental forces. Moreover, recent advances in quantum teleportation are demonstrating that the seemingly impossible might soon become feasible. These discoveries reshape our concept of reality, challenging what we thought we knew about space, time, and matter.
How Recent Quantum Breakthroughs Are Reshaping Our Understanding of Reality
Quantum breakthroughs have shifted our perception of the universe in fundamental ways. For instance, the concept of quantum entanglement—that particles can be linked regardless of distance—has been experimentally verified. These breakthroughs, including observations of superposition and quantum tunneling, are sending ripples through the physics community.
These findings reveal fundamental insights that bring us closer to understanding the nature of our universe. Every quantum experiment acts as a step toward answering the ultimate questions of ‘why’ and ‘how.’ The implications of these discoveries are not limited to theoretical physics; they are beginning to influence emerging technologies like quantum computers and ultra-secure communication networks.
In addition, these advancements have broad implications for our daily lives. Quantum discoveries are changing how we comprehend information, energy, and even the passage of time. The boundary between science fiction and science reality continues to blur, leading to technological applications that could revolutionize our world.
Key Fundamental Theories in Quantum Physics
Two of the most important theories in modern physics are quantum mechanics and quantum field theory. Together, they have laid the foundation for our understanding of atomic and subatomic processes.
Overview of Quantum Mechanics and Quantum Field Theory
Quantum mechanics describes the universe at the smallest scales—think electrons, photons, and other elementary particles. Quantum field theory takes this a step further, explaining how these particles interact through fields. Both theories are highly successful in their respective domains. However, they present challenges when attempting to reconcile them with classical theories like general relativity.
The mismatch between quantum physics and general relativity has puzzled scientists for decades. Each theory describes different aspects of reality with astonishing precision. However, unifying them into one coherent framework—often referred to as the Theory of Everything—remains one of the greatest challenges in physics.
This gap isn’t just theoretical; it also has practical implications. For instance, understanding quantum gravity could help us solve some of the greatest mysteries of the cosmos, such as the true nature of black holes or the singularity at the beginning of the universe. The quest for unity continues to drive scientists deeper into the quantum realm.
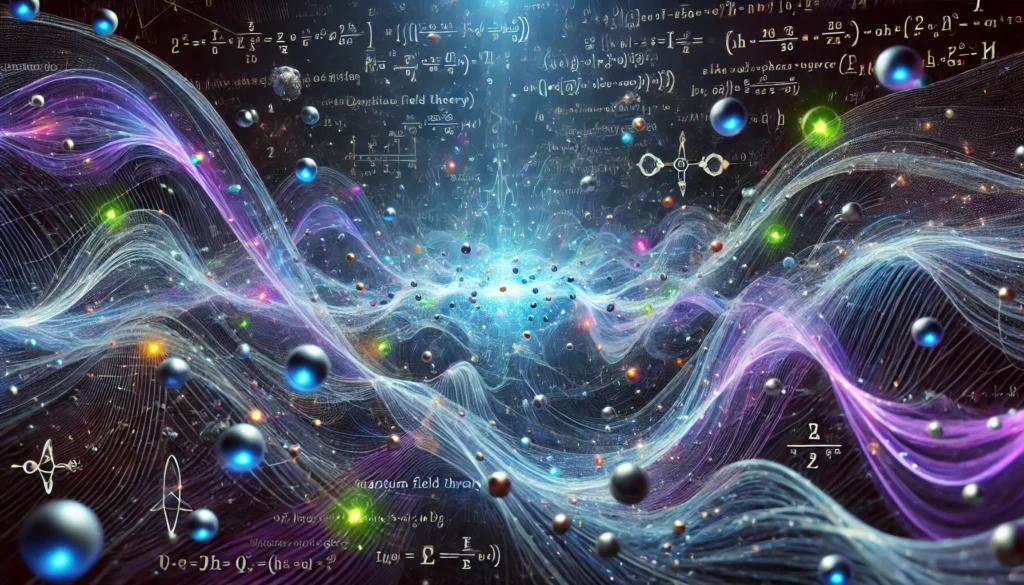
The Importance of Unifying Quantum Forces
In quantum physics, forces like electromagnetism, the strong force, and the weak force govern all interactions at the microscopic scale. These fundamental forces shape the behavior of all particles.
The ultimate goal of quantum physics is to develop a cohesive framework that unifies all of these forces. A ‘Theory of Everything’ would explain how forces and particles interact seamlessly, providing a deeper understanding of the universe. Right now, quantum mechanics aligns well with three of these forces, but integrating gravity into this framework has proven elusive.
Scientists believe that unifying these forces could unlock profound insights into the fundamental nature of the universe. Such a breakthrough would potentially reveal new particles or forces, reshaping our current understanding and leading to entirely new fields of study.
Quantum Particles: The Building Blocks of the Universe
What makes up everything around us? The answer lies in quantum particles—quarks, leptons, and bosons.
What Are Quantum Particles?
Quantum particles are the smallest known building blocks of matter. They are like the smallest Lego bricks that form all matter—including us. These particles include quarks, leptons, and bosons, each playing a critical role in the composition of the universe. Quarks, for instance, form protons and neutrons, which are found in the nucleus of atoms. Leptons, such as the electron, interact to form stable atoms as we know them.
Furthermore, quantum particles have no internal structure—they cannot be divided further. This indivisibility is what makes them fundamental to our understanding of nature. Their interactions are governed by quantum mechanics, which helps us understand their complex behavior and the forces that act upon them.
Moreover, each quantum particle interacts differently with the fundamental forces. This knowledge allows physicists to unravel the mysteries of how forces like electromagnetism and the weak force affect matter, leading to the creation of everything in our universe.
The Significance of Particles Like Quarks, Leptons, and Bosons
Quarks and leptons are the foundational particles that make up all matter. However, bosons—such as photons, gluons, and the Higgs boson—are crucial for mediating the forces that act upon quarks and leptons.
The discovery of the Higgs boson was a monumental milestone for quantum physics. It confirmed a key prediction of the Standard Model, specifically explaining why particles have mass. Without the Higgs field, elementary particles like quarks and leptons would remain massless, which means no matter as we know it would exist.
Bosons are the mediators of quantum forces. For example, photons are responsible for electromagnetism, while gluons mediate the strong force that binds quarks together in protons and neutrons. The Higgs boson provides a unique mechanism that gives mass to particles, tying theory to observable phenomena and greatly advancing our understanding of the quantum world.
The Quantum Standard Model: What It Explains and Its Limitations
The Quantum Standard Model is our best framework for understanding particle physics. It explains how particles interact and which forces influence them. However, it also has limitations.
Overview of the Quantum Standard Model
The Quantum Standard Model is incredibly successful at explaining three of the four known forces: electromagnetism, the strong force, and the weak force. However, it completely ignores gravity, which is essential for understanding large-scale structures such as planets, stars, and galaxies.
Additionally, the Standard Model does not explain dark matter and dark energy. These components make up about 95% of the universe, yet we lack any concrete understanding of their nature. Dark matter is thought to contribute to galaxy formation, while dark energy drives the universe’s accelerating expansion.
Therefore, while the Standard Model is powerful, it remains incomplete. Physicists are actively searching for new theories or new particles that could bridge these gaps, offering a more complete understanding of the universe’s underlying structure.
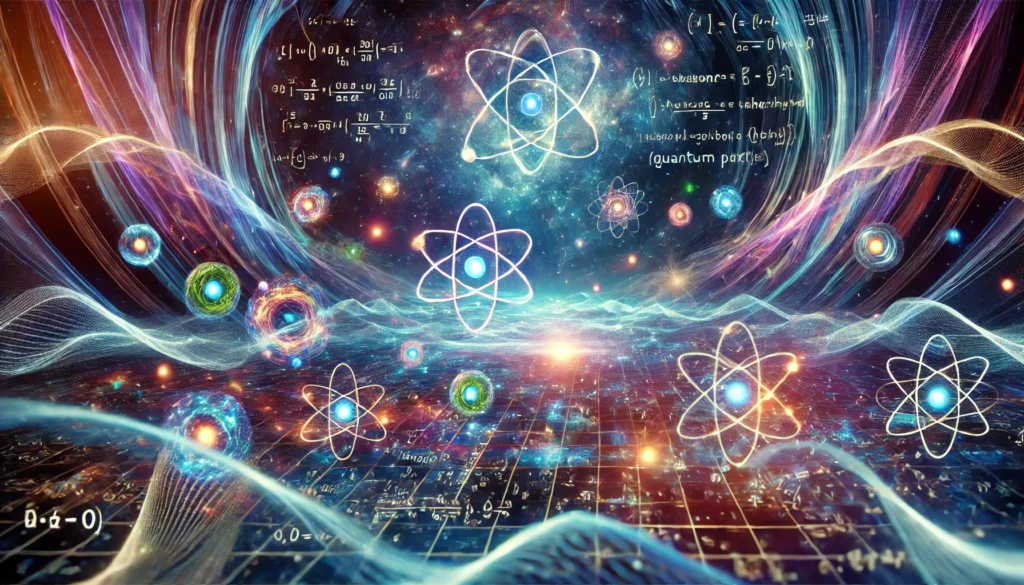
Searching for ‘New Physics’
Physicists are in pursuit of ‘new physics’—something that extends beyond the Standard Model. They are investigating theories such as supersymmetry and string theory, both of which attempt to address the limitations of the Standard Model.
Moreover, the Quantum Standard Model does not provide answers for dark matter, dark energy, or gravity. Finding a more complete theory might mean uncovering new particles or forces that we haven’t yet observed. Researchers believe that these discoveries could revolutionize our understanding of nature.
This ongoing effort is not merely academic; it aims to bridge fundamental gaps and potentially transform our technology and perception of the cosmos. Scientists continue to push the boundaries of quantum physics, seeking a unified framework that could describe all known physical phenomena.
The Role of Quantum Mechanics in Recent Breakthroughs
It isn’t just a theoretical curiosity; it’s the foundation of many groundbreaking technologies—and the breakthroughs keep coming.
Quantum Entanglement and Experimental Progress
It is the fascinating concept that particles can become linked, even when separated by vast distances. This idea, once thought impossible, has now been verified through a number of experiments. Entangled particles remain correlated in their properties no matter how far apart they are, offering new avenues for technology.
Quantum entanglement has practical applications that go far beyond theory. For example, it forms the basis of quantum cryptography, where secure communication is achieved through entangled states. If an eavesdropper attempts to intercept the communication, the quantum state will change, making the interception detectable.
Entanglement is also central to the development of quantum computers. It enables qubits to interact in complex ways, providing computational power far beyond that of classical computers. With continued progress, entangled quantum systems may reshape computation and information technology as we know it.
Advances in Quantum Computing and Their Impact
Quantum computers have made impressive strides, providing capabilities beyond anything possible with traditional computers. They offer solutions to problems that are practically unsolvable for classical computing systems. This includes optimizing complex systems, modeling molecules in chemistry, and tackling cryptographic algorithms.
The strength of quantum computing lies in qubits, which can exist in multiple states simultaneously. Unlike classical bits, which are either 0 or 1, qubits can represent both states due to superposition. Additionally, quantum entanglement allows qubits to perform computations in parallel, exponentially increasing computational power.
These advancements are not only theoretical but have potential real-world applications. Quantum computing could revolutionize industries such as medicine, cybersecurity, and artificial intelligence. Although quantum computers are still in development, their potential impact is immense, promising to push the boundaries of what technology can achieve.
Quantum Experiments at the LHC
The Large Hadron Collider (LHC) is the world’s most powerful particle collider, crucial for understanding quantum interactions.
How the LHC Contributes to Quantum Physics
The LHC accelerates particles to near-light speed and then collides them to create extreme energy conditions. These conditions resemble those just after the Big Bang, helping scientists understand the early moments of the universe.
Moreover, these high-energy collisions produce rare subatomic particles that provide insights into quantum fields and interactions. By analyzing the debris from these collisions, physicists can gain a deeper understanding of the universe’s building blocks and test predictions of quantum theories.
These experiments not only confirm existing models but also push our understanding to new limits, leading to questions that challenge our current theories. By doing so, the LHC helps to expand our understanding of quantum phenomena and the universe itself.
Key Discoveries at the LHC
In 2012, the LHC made its most famous discovery: the Higgs boson. This discovery confirmed the mechanism that gives particles mass, a crucial part of the Standard Model. But the LHC’s discoveries extend far beyond the Higgs.
Researchers have used the LHC to identify new composite particles and better understand quantum chromodynamics, the theory of the strong interaction. The collider has also helped scientists explore potential candidates for dark matter, providing valuable data that refines our theories.
Ongoing experiments at the LHC continue to push boundaries, offering new insights that challenge our understanding of quantum physics and open doors to potential new discoveries. The LHC remains a critical tool in the quest to understand quantum phenomena.
Advances in Understanding Dark Matter and Dark Energy Through Quantum Physics
Dark matter and dark energy are two of the most significant mysteries in physics today.
What Is Dark Matter, and Its Quantum Significance?
Dark matter does not emit light or energy, making it invisible and difficult to detect directly. However, quantum physics allows us to infer its presence by observing gravitational effects on visible matter. These gravitational effects influence galaxy rotation speeds and the movement of galaxy clusters.
Dark matter is crucial for the formation and stability of galaxies. Without it, galaxies would lack the gravitational pull necessary for their current structure. Quantum field theory suggests that dark matter might interact weakly with normal matter, offering a way to potentially detect it through particle collisions.
Dark matter plays a significant role in the universe’s large-scale structure, and understanding it would be a major leap in quantum physics. Detecting dark matter could help explain how quantum particles contribute to the universe’s formation and evolution.
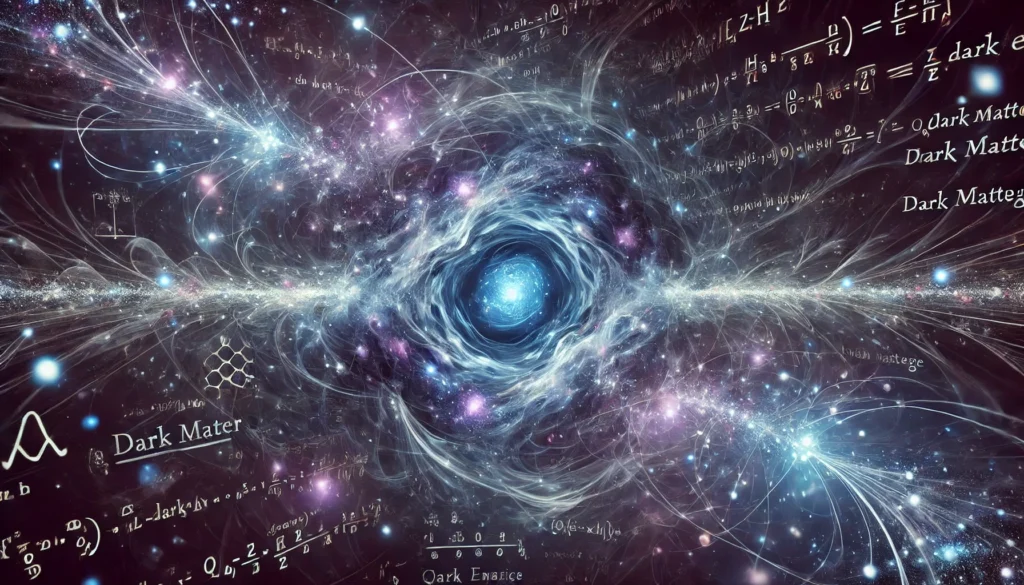
Current Experiments and Quantum Hypotheses
Several experiments are aimed at detecting dark matter through quantum interactions. Researchers use highly sensitive detectors, often located deep underground, to identify rare interactions between dark matter and ordinary matter.
By studying particle collisions, such as those produced at the LHC, scientists hope to create or detect dark matter particles. These experiments could provide evidence to understand this elusive component of the universe.
Moreover, discovering dark matter would be a groundbreaking achievement. It would reveal the nature of the invisible portion of cosmic matter and help solve one of quantum physics’ most perplexing puzzles.
Challenges in Reconciling Quantum Physics with General Relativity
Quantum physics and general relativity are both cornerstones of our understanding, but they don’t fit together easily.
Why Quantum Physics and General Relativity Remain Incompatible
Quantum physics explains phenomena at the smallest scales, like atoms and subatomic particles. On the other hand, general relativity explains gravity and the behavior of massive objects, such as stars and galaxies.
However, applying quantum rules to gravity creates inconsistencies. The mathematical models that successfully describe quantum particles fail when extended to gravitational forces, leading to unsolvable equations.
This incompatibility highlights the need for a new theory that unifies these two domains. Physicists hope to discover a quantum theory of gravity, potentially solving mysteries like black holes or explaining the very beginning of the universe. The search for a unifying theory continues, driving research into new and exciting realms of physics.
The Quest for a Unified Quantum Theory
Physicists are striving for a unified theory—often called the Theory of Everything—that combines quantum mechanics and general relativity. String theory is one of the leading candidates, proposing that the fundamental particles are actually tiny strings that vibrate at specific frequencies.
String theory also introduces the concept of additional spatial dimensions, which could explain how gravity fits with the other fundamental forces. Despite its potential, experimental verification remains challenging, and string theory has yet to provide conclusive answers.
Other approaches, like loop quantum gravity, offer alternative solutions to the problem of unification. Despite ongoing research, a unified quantum theory remains elusive, but physicists are determined to find a solution that brings together all known forces and particles.
The Future of Quantum Physics: Emerging Theories and Questions
The journey of discovery in quantum physics is far from over. In fact, it’s just beginning.
Emerging Quantum Theories and Experiments
Future quantum experiments, such as those involving larger particle accelerators, promise to push the boundaries of our knowledge even further. Proposed projects like the Future Circular Collider will provide unprecedented energy levels for exploration, revealing new details about quantum interactions.
Researchers are also focused on discovering new particles that could explain phenomena like dark matter. Furthermore, understanding dark energy remains a major priority, as it is responsible for the universe’s accelerated expansion.
Reconciling quantum mechanics with gravity remains a monumental challenge. However, the pursuit of a unified theory drives quantum research forward, promising to answer questions that have long puzzled physicists and open new possibilities for understanding the universe.
Fundamental Questions on Quantum Physics Awaiting Answers
How did the universe originate, and what sparked the creation of time and space? What are the true building blocks that constitute everything in existence, from atoms to galaxies? These questions are at the heart of quantum research today.
Answering them could lead to a unified theory that explains all known particles and forces. This would be an enormous leap in our understanding of the cosmos, potentially transforming science and technology in ways we can’t yet imagine.
Ultimately, the quest to answer these questions is what makes quantum physics so thrilling. It isn’t just about understanding nature—it’s about redefining it, finding new ways to describe reality, and pushing the boundaries of what we know is possible.
FAQ about Quantum Physics
What Are Some Recent Breakthroughs in Quantum Physics?
What Is the Quantum Standard Model?
How Does the LHC Contribute to Quantum Physics?
What Is the Role of Quantum Mechanics in Modern Physics?
What Are the Fundamental Quantum Forces?
What Are Current Theories About Dark Matter and Dark Energy?
How Does the Higgs Boson Fit Into the Quantum Model?
Why Is It Difficult to Unify Quantum Physics with General Relativity?
What Is the Future of Quantum Research?
How Do Particle Accelerators Work?
Conclusion
Quantum physics is always evolving. It’s filled with more questions than answers—and maybe that’s why it’s so captivating. Each new experiment pulls back the curtain a little more. While the universe isn’t giving up its secrets easily, quantum physics brings us closer to understanding reality with every discovery.